New technologies affecting reliability
Журнал: Научный журнал «Студенческий форум» выпуск №31(82)
Рубрика: Физико-математические науки
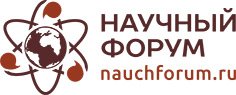
Научный журнал «Студенческий форум» выпуск №31(82)
New technologies affecting reliability
In recent years, a number of new technologies providing energy, capacity and other grid services have become available. The material below describes some of the most relevant technologies currently being deployed that may impact how reliability is considered in bulk power systems, in either a positive or negative way.
Large scale wind and solar power
According to the Global Wind Energy Council, there was 432.9 GW of wind generation installed worldwide by the end of 2015 (with more than 60 GW installed, 2015 was the highest year on record). Eight countries worldwide have more than 10 GW of installed capacity. By 2020, the Council forecasts 792 GW of wind power. Even if optimistic, it shows the large increase expected in the short term, and even more that may come online in the planning time frame.
Most renewable generation such as wind and solar cannot be fully dispatched-down; it must be used when available and where the power output varies with the weather. If communication and control is available, it can be dispatched-down if required, but there is often a large opportunity cost to do so, and many regions have requirements for a certain penetration of demand to be met by renewable sources. At least three characteristics of wind and solar lead to changes in system operation and planning:
1. Variability: wind and solar vary over time frames from seconds, minutes, hours, days and seasons. This uncertain variability complicates integrating these resources into plans and operation. Weather variability across these time frames requires that additional supply resources be available to provide power and energy when needed. In shorter time frames, this takes the form of increased operating reserve requirements. Experience shows that both wind and solar can increase the need for operating reserves by as much as 20% of installed capacity; though it can often be much lower. Generally, the amount required as a percentage of capacity increases with increased wind and PV penetration. By considering larger areas, diversity in wind and solar power output can help reduce the overall variability and thus reduce balancing requirements, assuming sufficient transmission exists to balance over the larger area.The variability inherent in wind and solar power also impacts resource reliability.
For wind power, seasonal variations in the output may mean that it is not always available during high-load periods; while solar, with its diurnal shape, may or may not line up with hours (or season) of peak demand. Thus, their contribution to generation reliability can be relatively limited. While wind power contributes to resource adequacy, it normally contributes a very low percentage to installed capacity. Solar power may contribute to systems with daytime peaks, but will not contribute to nighttime-peaking systems. Additionally, both wind and solar see a significant decrease in capacity contribution as penetration levels increase because their output is correlated, and thus, eventually move periods of highest loss-of-load risk to other periods of the day or year. Larger geographic areas typically see a higher capacity contribution from VNS renewables.
2. Uncertainty: Wind and solar, as well as being variable, also pose challenges in terms of predicting how that variability will occur. As with variability, this occurs in the same multiple time frames, from seconds to seasons. As weather cannot be perfectly predicted, the output of these resources is also only partly predictable.
Much progress has been made in the past decade on improving forecasting methods, particularly for wind but also more recently for solar. Typically, forecasts are based on large-scale numerical weather prediction models, which are then used with local sensors, satellite models, and statistical techniques, to predict wind and solar power output at suitable time horizons (hour-ahead, day- ahead, etc.).
As with variability, increasing the area under consideration can improve forecast accuracy. Typical values for forecast error are 10% root mean square error for a single wind farm, but only 4%-5% at larger levels such as over the German system. From a reliability perspective, this requires having sufficient flexibility to manage such forecast errors, either through conventional generation, storage, or demand-side options. Additionally, increased interaction with neighboring regions helps mitigate uncertainty.
3. Inverter Interface: Most modern wind plants, and all solar plants, interface to the electric power system using inverters to convert from DC to AC. They are designed to ‘follow’ system frequency and do not inherently respond to a frequency disturbance unless explicitly controlled to do so. Further, due to their near-zero marginal operating cost, PV and wind generation are almost always operated to produce maximum output, with the result that they have no "headroom” for responding to under-frequency events.
Most manufacturers of inverter-connected wind generators offer inverter control packages that can emulate inertial response by borrowing kinetic energy from the rotating mass to inject additional real power to the system, but the response is limited and must be “paid back” within a few seconds to prevent the turbine from stalling. These capabilities are not typically included unless required by their interconnection agreement.
Both wind and solar generators can provide primary frequency response if controlled to do so; for over-frequency response, this is well tested and used in many areas. Under-frequency response requires some curtailment, and, while not economically desirable, has also been shown to be a very effective resource when called upon. (For example, in ERCOT, under-frequency response is required from wind when it is already curtailed and has been shown to work well).
Other characteristics of wind and solar power may also impact on reliability. For example, much of the wind power is located far from the load and thus may not provide local support, and will require significant transmission construction to access the resource. As discussed in the next section, much of the PV generation may be distributed, bringing significant challenges.With increased penetrations of wind and solar, revenue sufficiency may become a serious issue.
Even with increasing wind and PV penetration conventional generation is still required to meet peak demand and provide other services (at least until energy storage and demand-side options become more widely available). As this new renewable generation reduces conventional generation output, there will be less revenue for conventional generation, possibly enough that they are no longer economically viable. Unless they are compensated in some other way their retirement may jeopardize system reliability.
Battery energy storage
The primary use of energy storage has been to store energy during periods when energy demand and/or costs/prices are low, and release it when demand and/or costs/prices are high. Energy storage, mainly in the form of pumped-hydro storage, has been used to provide this arbitrage for decades, and is well established. In most cases, this lowers system-operating costs, reduces start/stop operations of conventional generation and increases revenues for plant owners. With increasing levels of variable generation, the potential for arbitrage may increase in many systems, thus improving the economic outlook for storage. In some cases, without storage, large amounts of wind or solar may be curtailed during low-load periods. In these cases, adding storage allows their output to be stored for release later when load is higher or their output is low. As renewable generation curtailments increase, the economic benefits of adding storage will outweigh the costs by allowing more use of these resources.
Batteries being installed today are usually energy limited (typically less than 2 hours of energy at maximum output at present). Thus, they can only make a relatively small contribution to resource adequacy. In the future, longer duration batteries may also start to become more prevalent as costs of storing energy come down and new battery chemistries are used. In many areas, a specified number of continuous hours of availability are required before being allowed to contribute as an adequacy resource (e.g. in many US ISOS, 4 hours is needed). Therefore, longer duration batteries may be able to better contribute to resource adequacy. With increasing renewables, and their increased capacity contribution with storage, they should become more relevant during peak-demand periods.
Batteries are also ideally suited for many other reliability services. For example, their quick and accurate response means they can contribute to frequency regulation and spinning reserves. In many cases, they are more accurate than conventional resources. In the US, FERC has mandated the ISOS to reward frequency regulation resources, such as batteries, that can respond more accurately. Some system operators use different frequency regulation signals for batteries that use both their speed and accuracy, as well as recognizing their energy limits. Due to their relatively limited energy, batteries are probably best suited to this quick response rather than longer tertiary reserve uses. As well as contributing to bulk system services, batteries can also help with local issues. Distributed energy storage can help mitigate local impacts of rooftop PV, for example, microgrids can be aided significantly by batteries when operating in islanded mode.
In conclusion еach equipment has its positive aspects, but there are a sufficient number of disadvantages, over which work is underway to reduce their number or eliminate. Many technologies are expected to become more prevalent in coming years, and so are described here in terms of the characteristics that may have an impact on measuring bulk power system reliability.
